BCL11A-based gene therapy for sickle cell disease passes key preclinical test
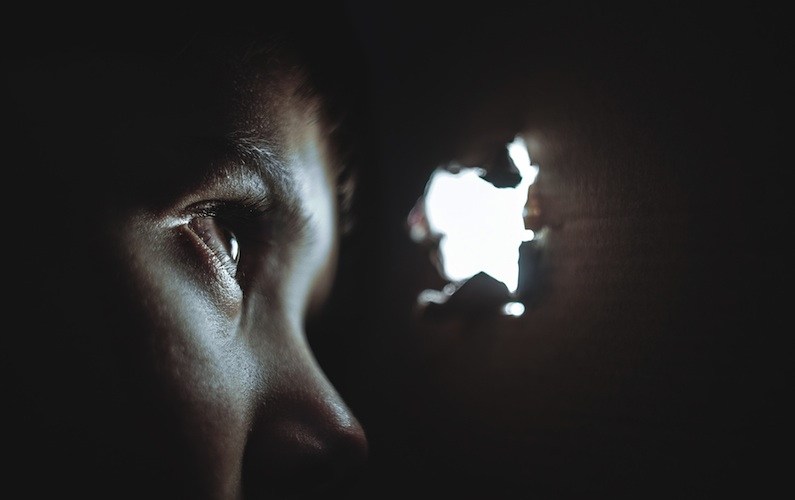
Research going back to the 1980s has shown that sickle cell disease is milder in people whose red blood cells carry a fetal form of hemoglobin. The healthy fetal hemoglobin compensates for the mutated “adult” hemoglobin that makes red blood cells stiffen and assume the classic “sickle” shape.
Normally, fetal hemoglobin production tails off after birth, shut down by a gene called BCL11A. In 2008, researchers Stuart Orkin, MD, and Vijay Sankaran, MD, PhD, at the Dana-Farber/Boston Children’s Cancer and Blood Disorders Center showed that suppressing BCL11A could restart fetal hemoglobin production; in 2011, using this approach, they corrected sickle cell disease in mice.
Now, the decades-old discovery is finally nearly ready for human testing — in the form of gene therapy. Today in the Journal of Clinical Investigation, Dana-Farber/Boston Children’s researchers report that a precision-engineered gene therapy vector suppressing BCL11A production overcame a key technical hurdle.
Reducing sickling in red blood cells
A red blood cell’s tendency to sickle is proportional to how much non-sickling versus sickling hemoglobin it has, says Williams. Gene therapy to silence BCL11A both increases fetal hemoglobin, which does not lead to sickling, and suppresses the beta (adult) hemoglobin affected by the sickle cell mutation.
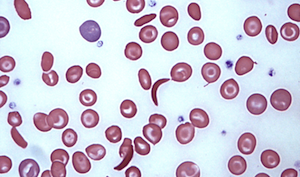
To get gene therapy into red blood cells, the corrective elements must first be inserted into blood stem cells that are transplanted into the body. These cells then start to create specialized blood cells, including red blood cells able to make fetal hemoglobin.
But the team, led by Dana-Farber/Boston Children’s researchers David A. Williams, MD, Christian Brendel, PhD, and Swaroopa Guda, PhD, discovered a major problem when doing tests in mice with the sickle cell mutation: when BCL11A is silenced, the transplanted blood stem cells cannot engraft long-term in the animals’ bone marrow and eventually become depleted. This was a major pitfall — one that could render gene therapy ineffective and also seriously undercut blood development in general.
Precision engineering
Circumventing the problem required several layers of extra engineering of the gene therapy vector. The result was a vector that silences BCL11A selectively — only in precursors of red blood cells.
As the core of the vector, the researchers used a short hairpin RNA (shRNA), a snippet of genetic code that inactivates the BCL11A gene. To get the shRNA into cells, they embedded it in a microRNA, another bit of code that cells generally recognize and process. To make this assembly work in the right place at the right time, they hooked it to a promoter of beta hemoglobin expression, together with regulatory elements active only in precursors of red blood cells.Finally, they inserted the whole package into a lentivirus engineered for safe use in humans. Blood stem cells from mice and the sickle-cell patients were then exposed to the virus, taking up the new genetic material. Upon transplantation, the treated cells successfully engrafted in the mice and began making blood cells. The red-blood-cell precursors, now genetically altered, began producing fetal hemoglobin rather than the mutated beta hemoglobin, just as intended.
In red blood cells from the mice and from four patients with sickle cell disease, fetal hemoglobin crowded out the sickling beta hemoglobin. Originally, to avoid clinical sickle cell disease, the researchers had hoped to get fetal hemoglobin to make up at least 50 percent of the cells’ hemoglobin. They far exceeded this goal, achieving fetal hemoglobin levels of at least 80 percent.
“The tendency for a red blood cell to sickle is proportional to how much non-sickling versus sickling hemoglobin it has,” says Williams, the study’s senior author and president of Dana-Farber/Boston Children’s.
Most importantly, the treated mice had reduced signs of sickle cell disease (hemolytic anemia and increased numbers of reticulocytes).
A double benefit
Other gene therapy trials for sickle cell disease are underway or are being developed. Rather than interfering with BCL11A, these approaches are introducing genes that encode fetal hemoglobin itself or a corrected beta hemoglobin that doesn’t sickle.
Williams believes the BCL11A-based approach to gene therapy for sickle cell disease will substantially increase the ratio of non-sickling versus sickling hemoglobin.
“BCL11A represses fetal hemoglobin, which does not lead to sickling, and also activates beta hemoglobin, which is affected by the sickle-cell mutation,” he says. “So when you knock BCL11A down, you simultaneously increase fetal hemoglobin and repress sickling hemoglobin, which is why we think this is the best approach to gene therapy in this disease.”
This approach, he adds, may also be beneficial in beta-thalassemia, another blood disorder involving defective beta hemoglobin.
Williams has launched multiple other gene therapy trials at Dana-Farber/Boston Children’s, which has become a world-leading hub of gene therapy for pediatric genetic diseases. His team is now taking the final steps toward FDA clearance for a clinical gene therapy trial in sickle cell disease that is expected to begin in early 2017.
Brendel and Guda are co-first authors on the paper. The work was supported by the Clinical and Translational Investigation Program at Boston Children’s Hospital, the National Institutes of Health (HL117720-03; DK093705 [NIDDK]), the Doris Duke Charitable Foundation and sponsored research support from Bluebird Bio.
If you are a physician with a complex-care patient to refer, call Boston Children’s priority line (1-844-BCH-PEDS).
Related Posts :
-
Tough cookie: Steroid therapy helps Alessandra thrive with Diamond-Blackfan anemia
Two-year-old Alessandra is many things. She’s sweet, happy, curious, and, according to her parents, Ralph and Irma, a budding ...
-
Unique data revealed just when Mickey’s heart doctors could operate
When Mikolaj “Mickey” Karski’s family traveled from Poland to Boston to get him heart care, they weren’t thinking ...
-
It’s all in the PV loops: New analytical model could improve circulation assessments before heart surgery
The double-switch operation corrects the congenital reversal of the heart’s ventricles and its two main arteries. It’...
-
Blood across our lifetimes: An age-specific ‘atlas’ tells a dynamic story
The stem cells that form our blood, also known as hematopoietic stem cells (HSCs), are with us throughout our lives. ...